Package Design Guide
This guide has been prepared to assist packaging designers in the selection and utilization of
ETHAFOAM brand polyethylene foam and other Performance Foams products for protective packaging applications. It contains recommended considerations and procedures for the design and development of highly protective and cost-efficient packaging.
This information is offered to facilitate the process of designing and developing packing that provides the desired level of protection at the most reasonable cost. It is recommended that, once a package design has been determined, a prototype product-package combination should be developed and tested for performance prior to final inspection.
Preliminary Design Considerations
The first step in designing a protective packaging solution is the selection of a cushioning material that is appropriate for the application.
As a general rule, ETHAFOAM polyethylene foams are best suited to protect products which can be described as relatively high in value and moderately fragile. Keeping damage rates for products of this nature to a minimum requires a strong, resilient cushioning material that reliably protects against multiple impacts. In other words, care must be taken to select a cushioning material that is not damaged by initial impacts and recovers to maintain its cushioning protection against repeated shocks.
ETHAFOAM products are resilient in nature, giving them outstanding recovery characteristics that provide outstanding cushioning protection against repeated impacts. And ETHAFOAM products are available in a variety of strengths, making them suitable for protecting products ranging from just a few pounds up to hundreds of pounds in weight.
TEP ONE: Determine Product Fragility
The first step toward determining the amount of cushioning a product requires is to determine the amount of mechanical shock the product can survive on its own. There are several common terms for this, with "fragility" and "g-factor" being the most common.
Fragility is normally expressed in units of "g’s" and indicates the maximum deceleration the product can withstand without being damaged. The more fragile a product is, the lower its g-factor. The table below helps to illustrate this concept.
Approximate Fragility of Typical Packaged Articles
Class | Typical Contents | Fragility |
Extremely Fragile | Missile guidance systems, precision aligned test instruments | 15-25 g’s |
Very Delicate | Mechanically shock-mounted instruments and electronic equipment (Shock mounts should be firmly secure prior to packaging. They are provided for in-service protection only.) | 25-40 g’s |
Delicate | Aircraft accessories, electric typewriters, cash registers and other electronically operated office equipment | 40-60 g’s |
Moderately Delicate | Television receivers, aircraft accessories | 60-85 g’s |
Moderately Rugged | Laundry equipment, refirgerators, appliances | 85-115 g’s |
Rugged | Machinery | 115 g’s and up |
Ideally, the fragility of a product is determined by subjecting it to a series of gradually more severe shocks (decelerations) in order to find the lowest severity impact that will damage the product. The highest deceleration, which did not cause damage, is then known to be the product’s g-factor.
It may be necessary to determine fragility levels for a product in various orientations, as it is not uncommon for a product to exhibit greater strength in one direction than another. Even very similar models should be tested individually as it’s seldom that fragility for one model may be safely assumed from another.
There is no substitute for fragility test data. Educated guesses as to a product’s fragility are often counterproductive to the design process. If the g-factor is estimated too high, and the product is unable to survive as much shock as anticipated, the packaging will be underdesigned and significant shipping damage is likely to occur. On the other hand, if the g-factor is estimated too low, and the product can actually withstand more shock than anticipated, the packaging will be overdesigned and unnecessarily expensive.
STEP TWO: Determine Conditions the Product Will Encounter
Once product fragility is determined, the packaging designer should next consider the handling and transportation environment the product will face.
To establish the amount of shock the product may encounter, it is necessary to determine the height from which the product may be dropped. Drop heights are generally established by the product’s weight, which usually reflect how the product will be handled.
The chart below shows typical drop heights for products of various weights, and may be used if you lack specific information about the handling likely to be encountered in your product’s particular distribution chain.
Typical Drop Heights
Weight Range (lbs) | Type of Handling | Drop Height (in) |
0-10 | 1 person throwing | 42 |
10-20 | 1 person carrying | 36 |
20-50 | 1 person carrying | 30 |
50-100 | 2 person carrying | 24 |
100-250 | Light equipment | 18 |
250+ | Heavy equipment handling | 12† |
†NOTE: Palletized products may receive drops of up to six inches.
STEP THREE: Calculate Fundamental Cushion Requirements
When product fragility (g-factor) and handling environment (drop height) have been determined, the procedure that follows can be used to determine the amount of functional cushioning material which will provide adequate protection for the packaged item.
By functional cushioning material, we mean that portion of the design which directly supports the load and functions to absorb shock during impacts. There may be additional material used in the design as well to connect functional cushioning parts together, facilitate pack assembly, etc. This procedure does not take into account such factors as the effect of the outer container or other effects that may take place inside the container.
Generally such effects are beneficial so that this procedure can confidently be used as a starting point to develop packaging that provides an assured level of protection and optimum cost-efficiency. To calculate functional cushioning needs it will be necessary to understand and use dynamic cushioning curves.
Using Dynamic Cushioning Curves: An Overview
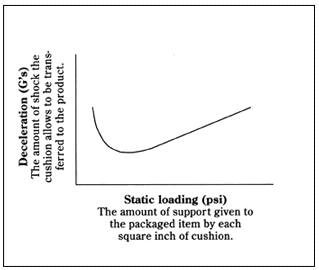 |
Typical dynamic cushioning curve |
A cushioning curve shows how a particular packaging material of a given thickness behaves at different impact conditions. Curves are generated by dropping a series of known weights onto a cushion sample from a specified height and measuring the amount of shock experienced by the weights as they impact the foam. In simple terms, this testing represents a product dropping on a cushion from a height likely to be encountered during shipment.
An idealized cushioning curve is shown below. It represents the cushioning performance of a cushioning material for a given combination of thickness and drop height. The horizontal axis represents a range of static loadings (in pounds per square inch) that packaged items might apply to the cushioning material. The vertical axis represents the shock experienced as the cushion is impacted. Curves are often presented for both first impact and multiple impact (average of drops 2-5) data. It is common to include data for several cushion thicknesses from a constant drop height on a single set of axes.
Cushioning curves for ETHAFOAM* products may be obtained by contacting your local Dow-authorized fabricator, your local Dow representative, or Dow’s Research and Development facility.
Using Dynamic Cushioning Curves:
An Example
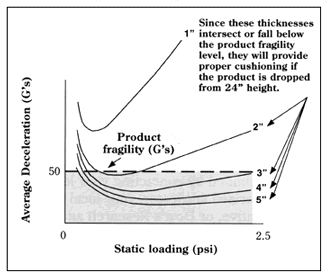 |
Determining Cushion Thickness 24" drop height, 2-5 drop average |
An object to be packaged is a 10-inch cube weighing 60 pounds with a fragility of 50 g’s. Since a product typically faces repeated impacts during shipment, you will probably wish to use multiple-impact data. The typical drop height for a product of this weight may be estimated from the chart below as 24 inches, however specific knowledge of shipping conditions or corporate standards may dictate a different choice for drop height.
First, obtain the cushioning curves for the cushioning material you wish to use. Locate curves that represent multiple impact data from a drop height of 24 inches. One such set of curves is shown here.
Determining Thickness
Using the chart below as a reference, locate our product’s fragility level (50 g’s) on the vertical axis of the figure, and draw an imaginary horizontal line across the chart at this level.
This separates the chart into two sections:
- our fragility line and lower, where the packaged item will be able to survive the anticipated shock level, and
- the section above our line where the shock levels are high enough to damage the product
Note that in this example, a 1-inch thickness of this cushioning material will not protect the item down to 50 g’s because the entire 1-inch curve is located above the 50 g line, in the "damage" zone. All of the other curves, representing cushions 2 inches thick and greater, have portions down in the "no damage" zone, and thus can be used. In most cases, shipping and handling considerations provide a strong cost incentive to design as small a package size as possible, so the thinnest cushion thickness which will do the job is most often selected. In this case, we will choose a thickness of 2 inches.
Determining Static Loading and Bearing Area
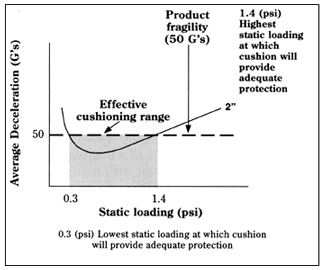 |
Determining Static Loading Range 24" drop height, 2-5 drop average |
Only a portion of the 2-inch cushion curve can be used to protect our product to 50 g’s. As seen below, the useable part of the curve is bounded by a static loading of 0.3 psi at the low end, and 1.4 psi at the high end. This tells us that, with a 2-inch cushion, we can apply a static loading anywhere within this "cushioning range" and still protect to 50 g’s or lower.
We now must choose a static loading to use within this range and design our cushions. The highest static loading value within the cushioning range will result in the most economical design because it will use less cushioning material to provide adequate protection, thus lowering design costs. Continuing with our example will illustrate how.
Once we have selected a static loading, we can calculate how many square inches of foam in our chosen thickness we’ll need to support and protect any side of the packaged product. In most cases it will be necessary to design a cushion to protect each of the faces of the product.
The cushion bearing area is easily calculated as the product weight divided by our chosen static loading. In this example, we have an allowable static loading range of 0.3 to 1.4 psi. If we were to choose to design with a static loading at the lower end of our range, 0.3 psi, we would divide our 60 pound product weight by 0.3 psi and find that we would need to support our product with 200 square inches of foam to produce a design which loads the foam to 0.3 psi.
If we were to choose the higher loading value of 1.4 psi instead, we would divide 60 pounds by 1.4 psi and find that only 43 square inches of foam are necessary to support the product at this higher loading. This is a 78.5% reduction in cushioning material compared to designing at 0.3 psi. You can imagine the cost savings that can result.
The use of higher loading values to optimize designs cannot be overemphasized. As a general rule, if you can double or triple the loading value and still not exceed your product fragility level, you can reduce your cushion usage by one-half to two-thirds. As a result, total material costs will be minimized.
Note that it may be possible to use even a smaller volume of foam by increasing thickness somewhat and taking advantage of the higher loading (and smaller bearing area) this allows. The resulting increase in shipping and handling costs due to package size increase is often greater than the saving in cushion material costs, so designing to the minimum thickness is the general practice.
By repeating this procedure with several materials, you can quickly generate comparisons, which will allow you to strike an economical balance between material cost and package size.
STEP FOUR: Recognizing Design Constraints
In most cases, the design approach we used in step three, i.e. using the highest possible static loading, will produce economical designs which adequately protect your packaged product. There are three constraints that must be checked however, to be sure this loading will not create other problems: compressive creep, cushion buckling, and extreme temperature effects.
Consider Compressive Creep
Compressive creep is the gradual loss of thickness a material may experience if placed under a constant load for an extended period of time. Significant compressive creep will result in the packaged product loosening in the cushion and becoming vulnerable to excessive movement inside the package during shipment.
As a general rule, creep of 10% is recognized as a practical upper limit. In some cases creep losses of over 10% in thickness have been shown to result in a significant loss of cushion performance.
Should it be found that creep in excess of 10% is anticipated, designers should recalculate the functional foam requirement using a lower static-loading figure. Spreading the loading over a larger area will reduce compressive creep.
The recommended loading limits to avoid excessive creep for various ETHAFOAM* polyethylene foam products are shown in below. Published dynamic cushioning data on ETHAFOAM cuts cushioning curves off at these limits to prevent you from inadvertently exceeding them.
These limits are believed to be adequate for temperatures encountered in a normal shipping environment. If exposure to excessive temperatures above 120°F are anticipated, lower loadings may be desirable. Consult product data sheets for compressive creep data on individual products.
Recommended Loading Limits for ETHAFOAM Brand Product
Material | Static Loading Limit |
ETHAFOAM Nova | 1.5 psi |
ETHAFOAM Select | 2.0 psi |
ETHAFOAM 220, 4101, M1 | 2.5 psi |
ETHAFOAM HS-45, M3 | 5.0 psi |
ETHAFOAM HS-600, M4 | 10.0 psi |
ETHAFOAM HS-900, M5 | 20.0 psi |
Consider Cushion Buckling
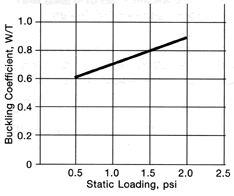 |
Buckling Coefficients for ETHAFOAM 220 at Various Static Loadings |
|
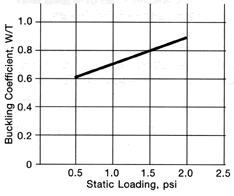 |
Buckling Coefficients for ETHAFOAM Select at Various Static Loadings |
|
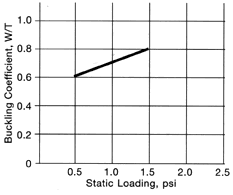 |
Buckling Coefficients for ETHAFOAM Nova at Various Static Loadings |
|
†Typical values, not product pecification limits. Note: for higher strenght, higher density ETHAFOAM products, the use of a constant buckling coefficient (W/T) of 0.75 is recommended. |
Buckling is the non-uniform compression of a cushion. When buckling occurs, the energy of the impact is not transferred evenly throughout the cushion and more shock is transferred to the package contents. Buckling usually occurs when the cushions become too tall and thin.
Buckling is actually less of a problem with ETHAFOAM* products than with many other cushioning materials. Yet it is one more factor you have to consider when designing a package. By using the charts and formulas presented below, you’ll be able to account for buckling in your designs and prevent any potential problems.
The tables help determine when the shape of the cushions can cause non-uniform compression, or buckling. They provide width-to-thickness coefficients for different static loading values and enable the designer to check for buckling potential.
To use the charts, determine the static loading (in psi) your product places on the cushion (divide product weight by surface area). Next, find the static loading value on the horizontal axis of the chart and read up to where the curve crosses it to find the buckling coefficient.
Next, multiply this coefficient by the thickness of the cushion to determine how wide the cushion must be to resist buckling. The example below will help illustrate how the chart and formula are used.
In cases where you must increase bearing area to avoid buckling, this results in decreased static loading. Check to see if this brings you into an appropriate loading range for the next lower strength grade of ETHAFOAM polyethylene foam, as it may be possible to redesign into a lower strength material more economically.
Let’s assume you plan to use a 3-inch thick pad of ETHAFOAM 220 to cushion a side of your product, which presents a static load of 10 psi. You’d refer to Buckling Coefficients for ETHAFOAM 220 at Various Static Loadings, find 1.0 psi along the bottom and follow the vertical line straight up until it intersects the plot line. From this point, follow the horizontal line to the left where you’ll find the W/T coefficient. In this case, the coefficient is 0.7. Using the formula T x W/T = W, you can now find the minimum width: 3"x 0.7 = 2.1". Your cushion must be at least 2.1 inches wide or long to resist buckling.
Note: For higher strength, higher density ETHAFOAM products, the use of a 0.75 buckling coefficient is recommended.
Consider Extreme Temperature Effects
One of the advantages of ETHAFOAM* polyethylene foams is that they retain their properties and characteristics with minimal change over a wide range of temperatures. However, as with all thermoplastic foams, when they are exposed to extremely high or low temperatures over a considerable length of time, they may be affected. The materials become stiffer at low temperatures and increasingly softer at higher temperatures. In extreme cases, it may become necessary to compensate for these effects in your design. Consult the individual product data sheets for ETHAFOAM available data on cushioning performance at extreme temperatures.
STEP FIVE: Design Prototypes and Test
Certainly no specific recommendations can be made about how to fabricate and position the foam on or around a particular product. Each packaging design must be developed separately, given the large number of variables involved.
The functional foam determinations made in conjunction with dynamic cushioning curves of ETHAFOAM* products, does not account for any protective contribution made by an outer container. It also does not account for effects such as shear and friction within the container, all of which may enhance the performance of the design to lower shock levels than those indicated by dynamic cushioning curves.
For this reason, it is highly recommended that you build and test a prototype of your cushion design to determine its actual performance. In many cases you will find that its performance exceeds your needs and, based on prototype testing, you can cut back on the amount of ETHAFOAM in the pack even further for an even more economical pack design. Any such modified designs should also then be tested to verify their performance.
Performance testing of prototype packages designed with ETHAFOAM polyethylene foam products is one of the services available from our research center. For more information, contact our technical staff at (800) 441-4369, or your local Dow-authorized fabricator.
STEP SEVEN: Monitor Performance
If it works, question it
There are many assumptions that went into your design development, among them that the drop height likely to be encountered is realistic, and that the fragility value used is representative of the product actually being packaged. Even when you are lucky enough to be working with a fragility value that was obtained by actual testing, in many cases what was tested was a prototype, and the production units may be more or less rugged than indicated.
For these reasons, it is a good practice to monitor the performance of your design in actual use. This will help you to determine if your design is providing more real-world performance than is actually required, or not enough. It can also allow you to determine when internal changes in the design of the packaged product might alter the requirements for package performance.
Just as no shipper can afford high damage rates, neither can he afford to overpackage. Monitoring of packaging performance on an ongoing basis can also help determine if further economies can be achieved without sacrificing protection.